Afr. J. Parasitol. Mycol. Entomol. , 2(1), 14; doi:10.35995/ajpme02020014
Insecticide Resistance in Malaria Vectors in Dielmo and Ndiop: Comparative Analysis of Knock Down Resistance Patterns using Two Sampling Methods in a Malaria Pre-elimination Context
1
Maladies Infectieuses, Négligées et Émergentes au Sud (MINES), IRD, AMU, Campus International IRD-UCAD, BP 1386, Dakar, Senegal; charles.bouganali@ird.fr (C.B.); diagnenafissatou51@gmail.com (N.D.); cheikh.sokhna@ird.fr (C.S.)
2
Laboratoire d’Ecologie Vectorielle et Parasitaire (LEVP), Faculté des Sciences et Techniques (FST), Université Cheikh Anta Diop (UCAD), Dakar, Senegal
3
Aix Marseille Univ, IRD, AMU, Maladies Infectieuses, Négligées et Émergentes au Sud (MINES), Marseille, France
*
Corresponding author: thiawomar185@yahoo.com (O.T.); souleymane.doucoure@ird.fr (S.D.); Tel.: +221-5175799 (O.T.); +221-5165485 (S.D.)
How to Cite: Thiaw, O.; Bouganali, C.; Diagne, N.; Doucouré, S.; Sokhna, C. Insecticide Resistance in Malaria Vectors in Dielmo and Ndiop: Comparative Analysis of Knock Down Resistance Patterns using Two Sampling Methods in a Malaria Pre-elimination Context. Afr. J. Parasitol. Mycol. Entomol., 2024, 2(2): 14; doi:10.35995/ajpme02020014.
Received: 16 October 2024 / Accepted: 25 October 2024 / Published: 28 November 2024
Abstract
:Introduction: Vector control is still confronted with the issue of insecticide resistance. This study investigates the impact of this phenomenon on entomological parameters and its distribution according to the mosquito sampling method in Dielmo and Ndiop, two malaria pre-elimination communities. Methods: Mosquitoes were sampled using human landing catch (HLC) and larval collection (LC) methods. Susceptibility tests were performed on LC samples. Larvae were collected using the dipping method from georeferenced breeding sites in the immediate vicinity of the study site. Five pyrethroids (alphacypermethrin, deltamethrin, lambdacyhalothrin, permethrin and etofenprox), one carbamate (bendiocarb) and one organophosphate (fenitrothion) were tested, using 2–5-day-old females. PCR techniques were used to identify Anopheles species and detect the Kdr mutation. Kdr frequencies were calculated under Hardy–Weinberg equilibrium and comparatively analyzed in terms of sampling method, biting behavior, longevity, vector species and study site, using a linear regression model. Results: Mosquitoes were fully susceptible to carbamates and organophosphates. For pyrethroids, mortality rates ranged from 80.3 to 100%. PCR revealed three species, An. coluzzii, An. gambiae and An. arabiensis. The latter predominated at both sites. Kdr frequencies ranged from 2.4 to 14% in Dielmo and from 17.3 to 40% in Ndiop. In Dielmo, An. arabiensis showed a low endophagic rate (36.6%). Biting behavior was independent of Kdr mutation, night period, species and mosquito lifespan but was dependent on the study site (p = 0.008). Comparing the sampling methods, there was a 43% lower chance of finding the kdr mutation in LC females (OR = 0.57; p = 0.03) in Dielmo, against a 3 times higher probability of detecting a Kdr allele in Ndiop (OR = 2.64; p = 0.001). Conclusion: This study underlines the importance of investigating insecticide resistance in host-seeking females, particularly in malaria pre-elimination settings.
Keywords:
malaria; An. gambiae s.l.; insecticide resistance; Dielmo; Ndiop; pre-eliminationIntroduction
The burden of malaria is ever-present in sub-Saharan Africa, which continues to pay the heaviest price, despite undertakings to progress towards the elimination of malaria in many countries [1]. Malaria prevention is largely based on effective vector control tools such as long-lasting insecticidal nets (LLINs) and indoor residual spraying (IRS). In 2020, 65% of people at risk were protected by LLINs and 2.6% by IRS [1]. Because of their low toxicity to humans and mammals and their high lethal effect on mosquitoes, pyrethroids are the main chemical class recommended by the World Health Organization (WHO) for treating bed nets [2]. However, the spread of pyrethroid resistance in malaria vector populations is threatening control strategies [1,3,4]. Intensive research has provided a description of a wide variety of insecticide resistance mechanisms in the main malaria vectors worldwide [5,6,7,8,9,10], among which is the knock down resistance (kdr) mutation, which undoubtedly confers resistance to pyrethroids and DDT [11]. On the other hand, malaria control could also be hindered by mosquito behavioral changes, which emerge as adaptive responses to implemented insecticide-treated tools [12,13,14]. To mitigate insecticide resistance, vector control research is generating new and promising approaches to control pyrethroid-resistant mosquitoes by manufacturing new generations of LLINs containing pyrethroids mixed with other insecticides that have different modes of action [15,16,17,18], or with synergist compounds [19,20,21,22], or even with insect growth regulators [23,24,25,26]. Faced with the challenges of the current context, assessing both insecticide resistance and vector behavioral adaptations should be the key components of control strategies in malaria endemic settings [27]. Measuring insecticide resistance in malaria vectors relies on bioassays, using either WHO insecticide susceptibility test kits or US Centers for Disease Control and Prevention (CDC) glass bottles, for mortality determination of adult mosquitoes against discriminating doses [28,29,30,31]. Most often, a random sub-sample of tested specimens, i.e., those from larval collections (LCs), is used for species identification and detection of resistance mechanisms such as the Kdr mutation [32,33,34,35,36,37,38,39,40]. However, as demonstrated in Anopheles sinensis, when using different sampling methods to assess insecticide resistance, a certain disparity often arises in resistance measurements [41]. The current malaria epidemiology, especially in pre-elimination areas, requires a careful analysis of the insecticide resistance issue; hence, the detection of the Kdr mutation should not only be restricted to a fraction of specimens used for bioassays but should also include indoor-resting [42,43] and host-seeking females.
In previous surveys carried out in Dielmo, western Senegal, the effect of malaria control tools on vector dynamics has been assessed [44], and a snapshot of the insecticide resistance profile has been taken, following nearly a decade of intense use of LLINs in this setting [32]. Dielmo and Ndiop are both in a malaria pre-elimination phase, and entomological surveillance activities, as well as insecticide resistance monitoring, are being performed in these villages.
This study aimed to update the insecticide susceptibility profile and make a comparative analysis of Kdr patterns in An. gambiae s.l., a major malaria vector in Dielmo and Ndiop, using two sampling methods, larval collection (LC) and human landing catch (HLC).
Methods
Study sites. This study was conducted during the rainy season, in September 2016, in Dielmo and Ndiop (Figure 1), two sites in western Senegal, in the Fatick region, 280 km from Dakar. Dielmo and Ndiop are 5 km apart and were included in a research project on malaria epidemiology that lasted over 25 years [45,46]. A description of Dielmo village was detailed at the beginning of the project, in 1990 [45], as was that of Ndiop, which joined the project three years later [46]. Since then, the sociodemographic status of Dielmo and Ndiop has been updated on a fairly regular basis [46,47,48,49]. Serere and Wolof are still the dominant ethnic groups in Dielmo and Ndiop, respectively. The main activities of the villagers remain agriculture (millet, groundnuts, maize). However, market gardening is relatively important in Dielmo thanks to the presence of a small permanent river, the Nema. In Ndiop, a large part of the population is engaged in rice growing in flood-prone areas a few miles from the village.
Socio-economically, one of the most significant events in these villages was their electrification between 2012 and 2015, enabling the regular use of television and the installation of a millet mill for women. However, access to electricity has also been associated with malaria epidemic rebounds, especially among people spending much of the night outdoors watching television [50]. Vector control was implemented in 2008 and since then, only LLIN universal coverage has been strategically used both in Dielmo and Ndiop. LLIN universal coverage was renewed in 2011, 2014, 2016, 2019 and 2022. During the period of the present study, in 2016, Ndiop was fully covered with permethrin-impregnated mosquito nets (Olyset® Net) and Dielmo with deltamethrin-impregnated ones (PermaNet 2.0®).
Figure 1.
Map showing the location of the study sites (Dielmo and Ndiop) with breeding sites and the distinctive Nema river in Dielmo.
Figure 1.
Map showing the location of the study sites (Dielmo and Ndiop) with breeding sites and the distinctive Nema river in Dielmo.
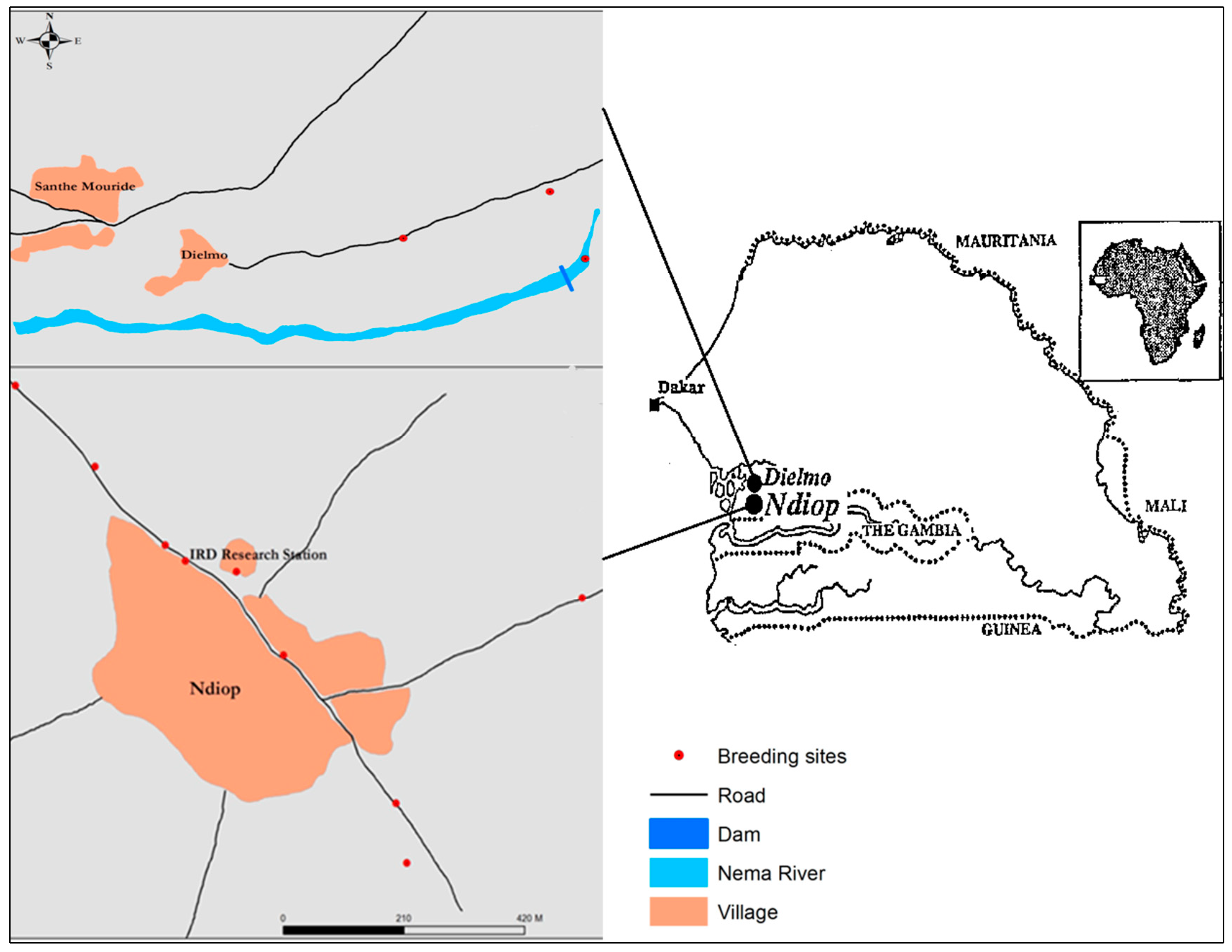
Field mosquito sampling and bioassays. Host-seeking mosquito females were captured both indoors and outdoors, from 7 p.m. to 7 a.m., using the human landing catch (HLC) method. Monthly captures were carried out over two consecutive nights in three rooms, using two volunteers per catching point, one inside and the other outside the room. The method consisted of catching females landing on the exposed body parts of the trapper by hypnotizing them with a torchlight before enclosing them in a hemolysis tube. Mosquito immature stages were collected in different breeding sites, as described in a previous study carried out in Dielmo [32]. Larvae were fed with a fish feed (TetraMin Baby®) and were maintained in a local insectary, where the temperature and the relative humidity conditions were controlled (25 ± 2 °C and 75 ± 10%). Pupae were collected daily and were transferred into crystallizers placed in cages covered with untreated mosquito nets. On emergence, adults were fed using a cotton swab soaked in a 10% sugar solution placed on the upper surface of the hatching cage. Larval collections (LCs) in the immediate vicinity of the study sites were coupled with HLC activities on the same monthly capture run, so that comparisons could be made between host-seeking females (HLC) and those collected from LCs. Captured host-seeking females were morphologically identified using dichotomous keys [51,52] and their ovaries were dissected for the determination of lifespan (longevity) through the calculation of the parity rates (ratio of the number of parous females to the total number dissected), as described by Detinova [53]. Susceptibility tests were carried out by exposing 3–5-day-old females from LCs to different discriminating doses of insecticides, using the Centers for Disease Control and Prevention (CDC, Atlanta GA) bottle bioassays, as described in a previous survey in Dielmo [32]. Insecticides were supplied by the CDC in hyperconcentrated formulations. In the laboratory, they were diluted in Falcon tubes by adding a volume of pure acetone (solvent) in order to obtain working solutions (50 mL) so that 1 mL of each corresponded to the diagnostic dose used to impregnate one bottle test. Control bottles were coated with 1 mL of solvent (pure acetone). Female mosquitoes were exposed for 30 minutes, which was the diagnostic time for all the insecticides used, including five pyrethroids (alphacypermethrin 12.5 μg/bottle, deltamethrin 12.5 μg/bottle, etofenprox 12.5 μg/bottle, lambdacyhalothrin 12.5 μg/bottle and permethrin 21.5 μg/bottle), one carbamate (bendiocarb 12.5 μg/bottle) and one organophosphate (fenitrothion 50 μg/bottle).
Mosquito laboratory processing. Mosquito genomic deoxyribonucleic acid (DNA) was extracted using CTAB method [32,54]. A random sub-sample of specimens from HLC and a batch of insecticide post-exposed females (consisting of randomly selected dead individuals and all survivors) was used for species identification and the detection of the Kdr mutation, as described by Wilkins et al. [55], and Hunyh et al. [56], respectively. Both Kdr-west (L1014F) and Kdr-East (L1014S) mutations were investigated. The steps in PCR procedures were detailed in a recent study [32].
Data analysis. The mortality rates of An. gambiae s.l. females exposed to insecticides were interpreted according to the WHO criteria, in which a 90% resistance threshold was defined. The allelic frequencies of the Kdr mutation (L1014F and L1014S) were calculated using the Hardy–Weinberg model. Wright’s fixation index (Fis) was determined and departures from the Hardy–Weinberg equilibrium were analyzed using Pearson’s Chi-2 test or Fisher’s test through the ‘HWExact’ function from the ‘GWASExactHW’ package in R software [57]. Means were compared using the Student test or the Kruskall–Wallis test, and 95% confidence intervals were calculated using the binomial exact test. Endophagic rates were determined as the ratio of the number of mosquitoes collected indoors (endophagous females) over the total captured using the HLC technique. A generalized linear model (GLM) analysis of indoor biting behavior was performed, where the odds ratio and 95% confidence intervals were calculated by negative binomial distribution, using the following explanatory variables: Kdr mutation, nocturnal biting behavior, Anopheles species, lifespan and study site. The mosquito nocturnal collection time was divided into two nighttime periods, which, for the purposes of analysis, will be referred to as “early” (7 p.m.–1 a.m.) and “later” (1–7 a.m.). GLM analysis of the Kdr mutation was also performed, using the sampling method (HLC or LC) and Anopheles species as explanatory variables.
Logistic regression analyses were performed using the ‘GLM’ function of R software. The significance level was set at 5%.
Results
Susceptibility of An. gambiae s.l. to insecticides. Bioassays were performed on a sample of 1070 specimens from Dielmo (n = 647) and Ndiop (n = 430). The results showed full susceptibility (100% mortality) to carbamates (bendiocarb) and organophosphates (fenitrothion) where these insecticides were tested (Table 1). Susceptibility to pyrethroids was relatively heterogeneous. Anopheles mosquitoes were fully susceptible (100% mortality) to lambdacyhalothrin and alphacypermethrin, which were tested only in Dielmo. However, a suspected resistance to etofenprox was noted, with respective mortality rates of 95.7% and 95.3% in Dielmo and Ndiop. Regarding deltamethrin and permethrin (Table 1, Figure 2), resistance was reported in Ndiop (87.5% and 80.3% mortality rates, respectively). However, a suspected resistance of An. gambiae s.l. to these latter pyrethroids was observed in Dielmo (95.2% and 92.5%, respectively). Molecular species identification indicated that the An. gambiae complex was represented by An. arabiensis, An. gambiae and An. coluzzii, with respective proportions of 74.66% (CI95%: 69.85–79,05), 12.36% (CI95%: 12.36–20.16) and 8% (CI95%: 6.57–12.84) in Dielmo and 76.28% (CI95%: 70.55–81.39), 9.49% (CI95%: 6.17–13.78) and 14.23% (CI95%: 10.17–19.15) in Ndiop (Figure 2).
Table 1.
Mortality rates of Anopheles gambiae s.l. from Dielmo and Ndiop following exposure to diagnostic doses of pyrethroid, carbamate and organophosphate insecticides.
Table 1.
Mortality rates of Anopheles gambiae s.l. from Dielmo and Ndiop following exposure to diagnostic doses of pyrethroid, carbamate and organophosphate insecticides.
Insecticides | Mortality Rates (%) | Mosquito Phenotype | ||
---|---|---|---|---|
Dielmo [CI95%] | Ndiop CI95%] | Dielmo | Ndiop | |
α-cypermethrin (97, 0) | 98 [92.7–99.7] | - | S | - |
Deltamethrin (83, 80) | 95.2 [88.1–98.7] | 87.5 [78.2–93.8] | R* | R |
λ-cyhalothrin (106, 93) | 100 [96.6–100] | 100 [96.1–100] | S | S |
Permethrin (94, 71) | 92.5 [82.3–97] | 80.3 [69.1–88.7] | R* | R |
Etofenprox (93, 86) | 95.7 [89.4–98.8] | 95.3 [88.5–98.7] | R* | R* |
Bendiocarb (83, 100) | 100 [95.7–100] | 100 [96.4–100] | S | S |
Fenitrothion (91, 0) | 100 [96.0–100] | - | S | - |
Mortality rates were interpreted using WHO criteria. Figures between brackets represent the total number of specimens tested for each insecticide in Dielmo and Ndiop. Figures between square brackets represent 95% confidence intervals for mortality rates. S, susceptible; R, resistant; R*, suspected of resistance.
Figure 2.
Species composition (A) and insecticide susceptibility status (B) in Dielmo and Ndiop. Solid and dotted horizontal bars indicate, respectively, susceptibility (98%) and resistance (90%) thresholds according to WHO criteria (WHO, 2016).
Figure 2.
Species composition (A) and insecticide susceptibility status (B) in Dielmo and Ndiop. Solid and dotted horizontal bars indicate, respectively, susceptibility (98%) and resistance (90%) thresholds according to WHO criteria (WHO, 2016).
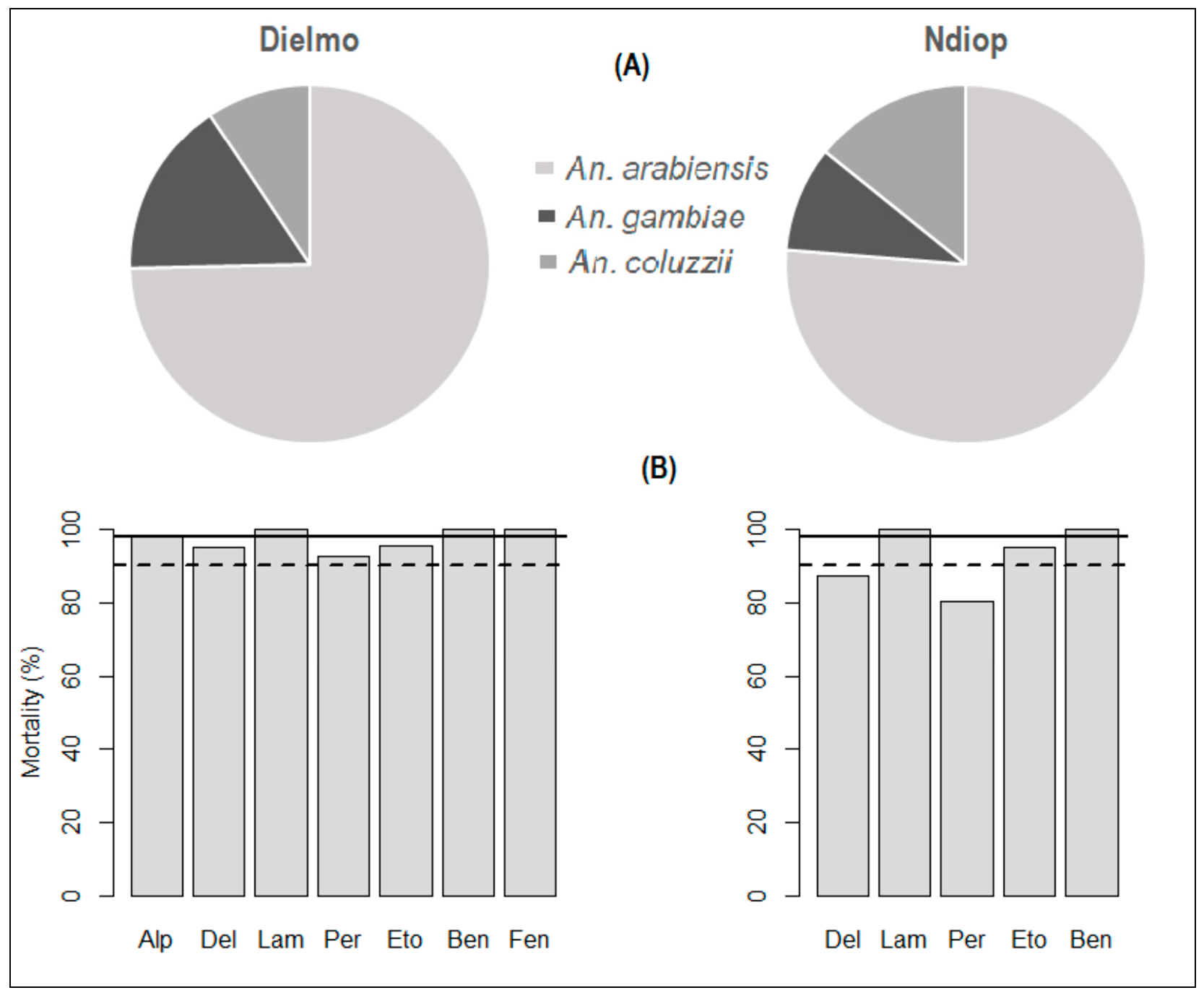
Kdr allelic frequencies under Hardy–Weinberg equilibrium. Kdr allelic frequencies were calculated by separating L1014F and L1014S mutations, thus focusing on a bi-allelic system approach. For An. arabiensis, An. coluzzii and An. gambiae, respectively, kdr-w (1014F) allele frequencies were 11.34%, 2.4% and 14% in Dielmo and 26.2%, 40% and 17.3% in Ndiop, while kdr-e (1014S) allele frequencies were 36.08%, 16.67% and 15.12% in Dielmo and 43%, 27.5% and 30.77% in Ndiop (Table 2). All An. arabiensis and An. gambiae populations presented heterozygote deficiencies, with fixation indices ranging from 0.692 to 0.821 (p < 0.05) in Dielmo and from 0.352 to 0.866 in Ndiop (p < 0.05), regardless of Kdr mutation type (Table 2). On the other hand, An. coluzzii populations showed an excess of heterozygotes in Dielmo (Fis = −0.024; p = 0.989) and Ndiop (Fis = −0.128; p = 0.003), when considering L10114F and L1014S mutations, respectively (Table 2).
Table 2.
Genotypes and Kdr-west (L1014F) and Kdr-east (L1014S) mutations in Dielmo and Ndiop under Hardy–Weinberg equilibrium.
Table 2.
Genotypes and Kdr-west (L1014F) and Kdr-east (L1014S) mutations in Dielmo and Ndiop under Hardy–Weinberg equilibrium.
Genotypes | 1014F/S | HWE | ||||||
---|---|---|---|---|---|---|---|---|
L1014F mutation | SS | RS | RR | Total | Freq. (%) | FIS (Wright) | P-value | |
An. arabiensis | Dielmo | 166 | 12 | 16 | 194 | 11.34 | +0.692 | <0.0001 |
Ndiop | 140 | 36 | 38 | 214 | 26.20 | +0.565 | <0.0001 | |
An. coluzzii | Dielmo | 20 | 1 | 0 | 21 | 2.40 | −0.024 | 0.989 |
Ndiop | 21 | 6 | 13 | 40 | 40.00 | +0.686 | <0.0001 | |
An. gambiae | Dielmo | 36 | 2 | 5 | 43 | 14.00 | +0.806 | <0.0001 |
Ndiop | 21 | 1 | 4 | 26 | 17.3 | +0.866 | <0.0001 | |
L1014S mutation | ||||||||
An. arabiensis | Dielmo | 116 | 16 | 62 | 194 | 36.08 | +0.821 | <0.0001 |
Ndiop | 88 | 68 | 58 | 214 | 43.00 | +0.352 | <0.0001 | |
An. coluzzii | Dielmo | 17 | 1 | 3 | 21 | 16.67 | +0.829 | <0.0001 |
Ndiop | 20 | 18 | 2 | 40 | 27.50 | −0.128 | 0.002 | |
An. gambiae | Dielmo | 35 | 3 | 5 | 43 | 15.12 | +0.728 | <0.0001 |
Ndiop | 16 | 4 | 6 | 26 | 30.77 | +0.639 | 0.003 |
HWE: Hardy–Weinberg equilibrium.
Impact of Kdr mutation on mosquito biting behavior. A mean endophagy rate of 36.6% (CI95%: 26.2–47.9) was observed in Dielmo (Table 3), indicating an outdoor biting behavior that particularly characterized An. arabiensis (binomial exact test: p = 0.02). In Ndiop, the mean endophagic rate was estimated to be 50.6% (CI95%: 42.7–58.5), showing a certain plasticity in biting activity, with a similar likelihood of indoor and outdoor biting behavior (binomial exact test: p = 0.94).
Multiple regression analysis demonstrated that indoor biting behavior was independent of Kdr mutation, nocturnal biting pattern (early or later), vector species (An. arabiensis versus An. gambiae and An. coluzzii) and longevity of host-seeking females. However, biting behavior was more influenced by the study site, with marked outdoor biting activity in Ndiop compared to Dielmo (p = 0.008) (Table 4).
Table 3.
Relative proportions (%) of three sibling species within Anopheles gambiae complex during indoor and outdoor human landing catches in Dielmo and Ndiop villages, Senegal.
Table 3.
Relative proportions (%) of three sibling species within Anopheles gambiae complex during indoor and outdoor human landing catches in Dielmo and Ndiop villages, Senegal.
Dielmo | Ndiop | |||||||||||
---|---|---|---|---|---|---|---|---|---|---|---|---|
Species | Indoor | Outdoor | Total | Indoor | Outdoor | Total | ||||||
ni | (%) | no | (%) | nt | (%) | ni | (%) | no | (%) | nt | (%) | |
An. arabiensis | 23 | (33.8) | 45 | (66.2) | 68 | (82.9) | 66 | (53.2) | 58 | (46.8) | 124 | (75.6) |
An. coluzzii | 3 | (37.5) | 5 | (62.5) | 8 | (9.8) | 13 | (41.9) | 18 | (58.1) | 31 | (18.9) |
An. gambiae | 4 | (66.7) | 2 | (33.3) | 6 | (7.3) | 4 | (44.4) | 5 | (55.6) | 9 | (5.5) |
Overall | 30 | (36.6) | 52 | (63.4) | 82 | (100) | 83 | (50.6) | 81 | (49.4) | 164 | (100) |
n(i, o, t), number of mosquitoes captured indoor (ni) and outdoor (no) on total collections (nt).
Table 4.
Generalized linear model (GLM) analysis of indoor biting behavior. Odds ratio and 95% confidence intervals were calculated by negative binomial distribution using the following explanatory variables: kdr mutation, nocturnal biting activity (time of night), species, parity and study site.
Table 4.
Generalized linear model (GLM) analysis of indoor biting behavior. Odds ratio and 95% confidence intervals were calculated by negative binomial distribution using the following explanatory variables: kdr mutation, nocturnal biting activity (time of night), species, parity and study site.
Indoor Biting | Odds Ratio | [CI95%] | p-Value | |
---|---|---|---|---|
Kdr mutation | RR vs SS | 0.754 | [0.413–1.364] | 0.353 |
Time of night * | Later vs Early | 1.270 | [0.730–2.224] | 0.402 |
Species | An. coluzzii vs An. arabiensis | 1.614 | [0.776–3.464] | 0.207 |
An. gambiae vs An. arabiensis | 0.845 | [0.271–2.624] | 0.767 | |
Parity | Parous vs Nulliparous | 1.344 | [0.283–7.106] | 0.708 |
Village | Dielmo vs Ndiop | 0.441 | [0.236–0.803] | 0.008 |
RR, homozygote resistant for Kdr mutation; SS, homozygote susceptible (Kdr-free); (*) time of night was divided into two periods: early (7 p.m.–1 a.m.) and later (1–7 a.m.)
Interactions between sampling method, Kdr mutation and Anopheles species. Generalized linear model (GLM) analysis revealed that the probability of encountering the Kdr mutation (in its heterozygous or homozygous form) varied significantly according to the sampling method (HLC vs. LC) and Anopheles species (Table 5). In Dielmo, there was an approximately 43% lower chance of finding the kdr allele in LC females compared to HLC females (OR = 0.57; p = 0.03). In contrast, in Ndiop, the probability of detecting individuals carrying the resistant allele was 3-fold higher in LC females compared to HLC females (OR = 2.64; p = 0.001). Likewise, the probability of finding the kdr allele was increased in An. coluzzii from Dielmo (OR =3.86; p = 0.007) and in An. gambiae from Dielmo (OR = 2.33; p = 0.012) and Ndiop (OR = 2.91; p = 0.019), compared to An. arabiensis.
Table 5.
Generalized linear model (GLM) analysis of Kdr mutation performed on 616 mosquitoes from Dielmo (N = 363) and Ndiop (N = 253) with odds ratio and 95% confidence intervals calculated by negative binomial distribution using sampling method (HLC or LC) and species as explanatory variables.
Table 5.
Generalized linear model (GLM) analysis of Kdr mutation performed on 616 mosquitoes from Dielmo (N = 363) and Ndiop (N = 253) with odds ratio and 95% confidence intervals calculated by negative binomial distribution using sampling method (HLC or LC) and species as explanatory variables.
Kdr Mutation | Site | Odds Ratio | [CI95%] | p-Value | |
---|---|---|---|---|---|
Method | LC vs HLC | Dielmo | 0.568 | [0.336–0.941] | 0.030 |
Ndiop | 2.636 | [1.443–4.914] | 0.001 | ||
Species | An. coluzzii vs An. arabiensis | Dielmo | 3.860 | [1.564–11.655] | 0.007 |
Ndiop | 1.460 | [0.594–3.370] | 0.387 | ||
An. gambiae vs An. arabiensis | Dielmo | 2.326 | [1.234–4.627] | 0.012 | |
Ndiop | 2.910 | [1.182–7.165] | 0.019 |
Kdr, knock down resistance; HLC, human landing catch; LC, larval collection.
Discussion
This study updates the insecticide resistance pattern of An. gambiae s.l. in Dielmo and Ndiop, two malaria pre-elimination settings. Compared to the first surveys, the overall trend has not changed for Dielmo, with persistently low Kdr frequencies (varying between 2% and 14% depending on the species). However, in Ndiop, where the insecticide resistance situation was not investigated during the first surveys, the Kdr frequencies were relatively high (17–40%). An outdoor biting behavior was observed in host-seeking mosquitoes, particularly at Dielmo.
In this study, three sibling species of the An. gambiae complex were encountered at the two study sites. Their respective populations generally showed a deviation from panmixia under the Hardy–Weinberg assumptions, with a generally closed reproductive pattern. The introduction of insecticide-treated mosquito nets was shown to have strongly influenced the genetic structure among Anopheles populations, with a degree of selection in favor of An. Arabiensis [1], which was the predominant species in our study. The phenotypic status of An. arabiensis towards insecticides did not change significantly compared to previous surveys [2], especially in Dielmo, where the Kdr mutation was still occurring at relatively low frequencies and with a suspected resistance to pyrethroids. In contrast, the situation was different in Ndiop, where pyrethroid resistance was confirmed, with higher kdr allelic frequencies compared to Dielmo. These disparities between Dielmo and Ndiop could be explained by some socio-economic and socio-anthropological factors. Indeed, agricultural activities were more developed in Ndiop, where the use of pesticides could induce a selection pressure and contribute to the increase in insecticide resistance. It is also worth noting that a study in Dielmo highlighted a higher malaria incidence among people who usually spent a good part of the night outside watching television [3]. This human behavior probably promoted some opportunism in host-seeking females, as expressed by the high exophagic rates recorded in this study. This outdoor biting behavior of host-seeking females was also consistent with the low kdr allelic frequencies observed in Dielmo, suggesting a low level of contact between An. arabiensis and insecticide-treated bed nets. Conversely, the mosquito indoor biting behavior observed in Ndiop was in line with the permethrin and deltamethrin resistance recorded in that site.
In this study, the Kdr mutation was estimated using two different sampling methods, i.e., larval collections (LCs), which targeted females immediately emerging from breeding sites (neonate females), and human landing catches (HLCs), focusing on host-seeking females taking action in households where insecticide-treated nets are used. The latter targeted population (HLC females) is of greater epidemiological significance and could provide Kdr frequencies that are probably more closely linked with the epidemiological picture, thus allowing for a better assessment of the impact of long-lasting insecticidal treated nets (LLINs) on the dynamics of vector populations and changes in their insecticide resistance patterns. This approach, which could be considered an entity of epidemiological entomology, as described by Killen et al. [4], allows for a better evaluation of the insecticide-based interventions put in place to fight malaria [5]. Comparisons between the LC and HLC “subpopulations” highlighted the existence of a certain selection of resistant genes under the effect of LLINs, with a higher probability of finding the kdr allele in host-seeking mosquitoes, presumably older females, compared to neonate ones, which would not yet have come into contact with LLINs. However, in our study, we did not find any causal link between the presence of the Kdr mutation and the nocturnal biting behavior of An. arabiensis, in the idea of establishing a correlation between the Kdr mutation and convenient vector biting time, particularly during the time when LLNs are used (later in the night), contrary to what was observed in an experimental study on An. gambiae s.s [6].
Conclusion
In the context of malaria pre-elimination, classical entomological investigation methods must be refined so as not to underestimate existing threats. In this study, the Kdr mutation was examined in both neonate and host-seeking females in Dielmo and Ndiop, two villages where impregnated mosquito nets have been the only vector control strategy since 2008. Our study highlights a particular interest in characterizing the resistance mechanisms of vector populations that present a direct link with malaria transmission in the context of pre-elimination and allows for a better understanding of data from standard methods used in the detection of insecticide resistance.
Author Contributions
O.T., S.D. and C.S. designed this study. S.D., N.D. and C.S. supervised this study. O.T., C.B. and S.D. carried out the field collections. O.T. performed the experiments, analyzed the data and wrote the manuscript. All authors have read and agreed to the published version of the manuscript.
Funding
This work was supported by the French National Research Institute for Sustainable Development (IRD), Campus UCAD/IRD de Hann, BP 1386. The funding body had no role in the design of this study, in the collection, analysis and interpretation of data and in the writing of the manuscript.
Acknowledgments
We thank the CDC for the supply of reagents and insecticides. We thank Hubert Bassene (IRD) for additional PCR reagents. We thank Elhadji Amadou Niang and Lassana Konaté (LEVP/UCAD) for helpful scientific advice. We are grateful to Dielmo and Ndiop villagers for their cooperation.
Conflicts of Interest
The authors declare that they have no competing interests.
References
- WHO. World Malaria Report 2021; World Health Organization: Geneva, Switzerland, 2021; Licence: CC BY-NC-SA 3.0 IGO. [Google Scholar]
- WHO. Malaria Control Today: Current WHO Recommendations; Working Document, March. Roll Back Malaria Department; World Health Organization: Geneva, Switzerland, 2005. [Google Scholar]
- WHO. World Malaria Report 2017; World Health Organization: Geneva, Switzerland, 2017; Licence: CC BY-NC-SA 3.0 IGO. [Google Scholar]
- WHO. World Malaria Report 2019; World Health Organization: Geneva, Switzerland, 2019; Licence: CC BY-NC-SA 3.0 IGO. [Google Scholar]
- Pavlidi, N.; Vontas, J.; Van Leeuwen, T. The role of glutathione S-transferases (GSTs) in insecticide resistance in crop pests and disease vectors. Curr. Opin. Insect. Sci. 2018, 27, 97–102. [Google Scholar] [CrossRef] [PubMed]
- Porciani, A.; Diop, M.; Moiroux, N.; KadokeLambi, T.; Cohuet, A.; Chandre, F.; Dormont, L.; Pennetier, C. Influence of pyrethroïd-treated bed net on host seeking behavior of Anopheles gambiae s.s. carrying the kdr allele. PLoS ONE 2017, 12, e0164518. [Google Scholar] [CrossRef] [PubMed]
- Wondji, C.S.; Dabire, R.K.; Tukur, Z.; Irving, H.; Djouaka, R.; Morgan, J.C. Identification and distribution of a GABA receptor mutation conferring dieldrin resistance in the malaria vector Anopheles funestus in Africa. Insect. Biochem. Mol. Biol. 2011, 41, 484–491. [Google Scholar] [CrossRef] [PubMed]
- Assogba, B.S.; Milesi, P.; Djogbenou, L.S.; Berthomieu, A.; Makoundou, P.; Baba-Moussa, L.S.; Fiston-Lavier, A.S.; Belkhir, K.; Labbe, P.; Weill, M. The ace-1 Locus Is Amplified in All Resistant Anopheles gambiae Mosquitoes: Fitness Consequences of Homogeneous and Heterogeneous Duplications. PLoS Biol. 2016, 14, e2000618. [Google Scholar] [CrossRef] [PubMed]
- Wood, O.; Hanrahan, S.; Coetzee, M.; Koekemoer, L.; Brooke, B. Cuticle thickening associated with pyrethroid resistance in the major malaria vector Anopheles funestus. Parasit. Vectors 2010, 3, 67. [Google Scholar] [CrossRef] [PubMed]
- Yahouedo, G.A.; Chandre, F.; Rossignol, M.; Ginibre, C.; Balabanidou, V.; Mendez, N.G.A.; Pigeon, O.; Vontas, J.; Cornelie, S. Contributions of cuticle permeability and enzyme detoxification to pyrethroid resistance in the major malaria vector Anopheles gambiae. Sci. Rep. 2017, 7, 11091. [Google Scholar] [CrossRef] [PubMed]
- Hancock, P.A.; Wiebe, A.; Gleave, K.A.; Bhatt, S.; Cameron, E.; Trett, A.; Weetman, D.; Smith, D.L.; Hemingway, J.; Coleman, M.; et al. Associated patterns of insecticide resistance in field populations of malaria vectors across Africa. Proc. Natl. Acad. Sci. USA 2018, 115, 5938–5943. [Google Scholar] [CrossRef] [PubMed]
- Gatton, M.L.; Chitnis, N.; Churcher, T.; Donnelly, M.J.; Ghani, A.C.; Godfray, H.C.; Gould, F.; Hastings, I.; Marshall, J.; Ranson, H.; et al. The importance of mosquito behavioural adaptations to malaria control in Africa. Evolution 2013, 67, 1218–1230. [Google Scholar] [CrossRef] [PubMed]
- Moiroux, N.; Gomez, M.B.; Pennetier, C.; Elanga, E.; Djenontin, A.; Chandre, F.; Djegbe, I.; Guis, H.; Corbel, V. Changes in Anopheles funestus biting behavior following universal coverage of long-lasting insecticidal nets in Benin. J. Infect. Dis. 2012, 206, 1622–1629. [Google Scholar] [CrossRef]
- Sougoufara, S.; Diedhiou, S.M.; Doucoure, S.; Diagne, N.; Sembene, P.M.; Harry, M.; Trape, J.F.; Sokhna, C.; Ndiath, M.O. Biting by Anopheles funestus in broad daylight after use of long-lasting insecticidal nets: a new challenge to malaria elimination. Malar. J. 2014, 13, 125. [Google Scholar] [CrossRef]
- N’Guessan, R.; Ngufor, C.; Kudom, A.A.; Boko, P.; Odjo, A.; Malone, D.; Rowland, M. Mosquito nets treated with a mixture of chlorfenapyr and alphacypermethrin control pyrethroid resistant Anopheles gambiae and Culex quinquefasciatus mosquitoes in West Africa. PLoS ONE 2014, 9, e87710. [Google Scholar] [CrossRef] [PubMed]
- N’Guessan, R.; Odjo, A.; Ngufor, C.; Malone, D.; Rowland, M. A Chlorfenapyr Mixture Net Interceptor(R) G2 Shows High Efficacy and Wash Durability against Resistant Mosquitoes in West Africa. PLoS ONE 2016, 11, e0165925. [Google Scholar] [CrossRef] [PubMed]
- Agossa, F.R.; Padonou, G.G.; Koukpo, C.Z.; Zola-Sahossi, J.; Azondekon, R.; Akuoko, O.K.; Ahoga, J.; N’Dombidje, B.; Akinro, B.; Fassinou, A.; et al. Efficacy of a novel mode of action of an indoor residual spraying product, SumiShield(R) 50WG against susceptible and resistant populations of Anopheles gambiae (s.l.) in Benin, West Africa. Parasit. Vectors 2018, 11, 293. [Google Scholar] [CrossRef] [PubMed]
- Mutunga, J.M.; Chen, Q.H.; Wong, D.M.; Lam, P.C.; Li, J.; Totrov, M.M.; Gross, A.D.; Carlier, P.R.; Bloomquist, J.R. Bivalent Carbamates as Novel Control Agents of the Malaria Mosquito, Anopheles gambiae. Chimia (Aarau) 2016, 70, 704–708. [Google Scholar] [CrossRef]
- WHO. Global Plan for Insecticide Resistance Management in Malaria Vectors; World Health Organization: Geneva, Switzerland, 2013. [Google Scholar]
- Moore, G.; Bingham, G. Use of “Temporal Synergism” to overcome insecticide resistance. Outlooks Pest Manag. 2005, 16, 7–9. [Google Scholar] [CrossRef]
- WHO. Conditions for Deployment of Mosquito Nets Treated with a Pyrethroid and Piperonyl Butoxide: Recommendations, September (Revised December); Global Malaria Programme; World Health Organization: Geneva, Switzerland, 2017. [Google Scholar]
- Toe, K.H.; Muller, P.; Badolo, A.; Traore, A.; Sagnon, N.; Dabire, R.K.; Ranson, H. Do bednets including piperonyl butoxide offer additional protection against populations of Anopheles gambiae s.l. that are highly resistant to pyrethroids? An experimental hut evaluation in Burkina Fasov. Med. Vet. Entomol. 2018, 32, 407–416. [Google Scholar] [CrossRef]
- Ngufor, C.; N’Guessan, R.; Fagbohoun, J.; Odjo, A.; Malone, D.; Akogbeto, M.; Rowland, M. Olyset Duo(R) (a pyriproxyfen and permethrin mixture net): an experimental hut trial against pyrethroid resistant Anopheles gambiae and Culex quinquefasciatus in Southern Benin. PLoS ONE 2014, 9, e93603. [Google Scholar] [CrossRef] [PubMed]
- Ngufor, C.; N’Guessan, R.; Fagbohoun, J.; Todjinou, D.; Odjo, A.; Malone, D.; Ismail, H.; Akogbeto, M.; Rowland, M. Efficacy of the Olyset Duo net against insecticide-resistant mosquito vectors of malaria. Sci. Transl. Med. 2016, 8, 356ra121. [Google Scholar] [CrossRef] [PubMed]
- Tiono, A.B.; Ouedraogo, A.; Ouattara, D.; Bougouma, E.C.; Coulibaly, S.; Diarra, A.; Faragher, B.; Guelbeogo, M.W.; Grisales, N.; Ouedraogo, I.N.; et al. Efficacy of Olyset Duo, a bednet containing pyriproxyfen and permethrin, versus a permethrin-only net against clinical malaria in an area with highly pyrethroid-resistant vectors in rural Burkina Faso: a cluster-randomised controlled trial. Lancet 2018, 392, 569–580. [Google Scholar] [CrossRef] [PubMed]
- Muema, J.M.; Bargul, J.L.; Nyanjom, S.G.; Mutunga, J.M.; Njeru, S.N. Potential of Camellia sinensis proanthocyanidins-rich fraction for controlling malaria mosquito populations through disruption of larval development. Parasit. Vectors 2016, 9, 512. [Google Scholar] [CrossRef] [PubMed]
- Killeen, G.F.; Marshall, J.M.; Kiware, S.S.; South, A.B.; Tusting, L.S.; Chaki, P.P.; Govella, N.J. Measuring, manipulating and exploiting behaviours of adult mosquitoes to optimise malaria vector control impact. BMJ Glob. Health 2017, 2, e000212. [Google Scholar] [CrossRef]
- Brogdon, W.; Chan, A. Guideline for Evaluating Insecticide Resistance in Vectors Using CDC Bottle Bioassay; CDC Stacks_Public Health Publications: Atlanta, GA, USA, 2012; 28p. [Google Scholar]
- Brogdon, W.G.; McAllister, J.C. Simplification of adult mosquito bioassays through use of time-mortality determinations in glass bottles. J. Am. Mosq. Control Assoc. 1998, 14, 159–164. [Google Scholar]
- WHO. Test Procedures for Insecticide Resistance Monitoring in Malaria Vector Mosquitoes; World Health Organization: Geneva, Switzerland, 2013. [Google Scholar]
- WHO. Global Malaria Programme: Test Procedures for Insecticide Resistance Monitoring in Malaria Vector Mosquitoes, 2nd ed.; World Health Organization: Geneva, Switzerland, 2016. [Google Scholar]
- Thiaw, O.; Doucoure, S.; Sougoufara, S.; Bouganali, C.; Konate, L.; Diagne, N.; Faye, O.; Sokhna, C. Investigating insecticide resistance and knock-down resistance (kdr) mutation in Dielmo, Senegal, an area under long lasting insecticidal-treated nets universal coverage for 10 years. Malar. J. 2018, 17, 123. [Google Scholar] [CrossRef] [PubMed]
- Betson, M.; Jawara, M.; Awolola, T.S. Status of insecticide susceptibility in Anopheles gambiae s.l. from malaria surveillance sites in The Gambia. Malar. J. 2009, 8, 187. [Google Scholar] [CrossRef] [PubMed]
- Djegbe, I.; Boussari, O.; Sidick, A.; Martin, T.; Ranson, H.; Chandre, F.; Akogbeto, M.; Corbel, V. Dynamics of insecticide resistance in malaria vectors in Benin: first evidence of the presence of L1014S kdr mutation in Anopheles gambiae from West Africa. Malar. J. 2011, 10, 261. [Google Scholar] [CrossRef] [PubMed]
- Namountougou, M.; Simard, F.; Baldet, T.; Diabate, A.; Ouedraogo, J.B.; Martin, T.; Dabire, R.K. Multiple insecticide resistance in Anopheles gambiae s.l. populations from Burkina Faso, West Africa. PLoS ONE 2012, 7, e48412. [Google Scholar] [CrossRef]
- Koffi, A.A.; Ahoua Alou, L.P.; Adja, M.A.; Chandre, F.; Pennetier, C. Insecticide resistance status of Anopheles gambiae s.s population from M’Be: A WHOPES-labelled experimental hut station, 10 years after the political crisis in Cote d’Ivoire. Malar. J. 2013, 12, 151. [Google Scholar] [CrossRef] [PubMed]
- Kerah-Hinzoumbe, C.; Peka, M.; Nwane, P.; Donan-Gouni, I.; Etang, J.; Same-Ekobo, A.; Simard, F. Insecticide resistance in Anopheles gambiae from south-western Chad, Central Africa. Malar. J. 2008, 7, 192. [Google Scholar] [CrossRef]
- Cisse, M.B.; Keita, C.; Dicko, A.; Dengela, D.; Coleman, J.; Lucas, B.; Mihigo, J.; Sadou, A.; Belemvire, A.; George, K.; et al. Characterizing the insecticide resistance of Anopheles gambiae in Mali. Malar. J. 2015, 14, 327. [Google Scholar] [CrossRef] [PubMed]
- Dia, A.K.; Gueye, O.K.; Niang, E.A.; Diedhiou, S.M.; Sy, M.D.; Konate, A.; Samb, B.; Diop, A.; Konate, L.; Faye, O. Insecticide resistance in Anopheles arabiensis populations from Dakar and its suburbs: role of target site and metabolic resistance mechanisms. Malar. J. 2018, 17, 116. [Google Scholar] [CrossRef]
- Zoh, D.D.; Ahoua Alou, L.P.; Toure, M.; Pennetier, C.; Camara, S.; Traore, D.F.; Koffi, A.A.; Adja, A.M.; Yapi, A.; Chandre, F. The current insecticide resistance status of Anopheles gambiae (s.l.) (Culicidae) in rural and urban areas of Bouake, Cote d’Ivoire. Parasit. Vectors 2018, 11, 118. [Google Scholar] [CrossRef]
- Xu, T.; Zhong, D.; Tang, L.; Chang, X.; Fu, F.; Yan, G.; Zheng, B. Anopheles sinensis mosquito insecticide resistance: comparison of three mosquito sample collection and preparation methods and mosquito age in resistance measurements. Parasit. Vectors 2014, 7, 54. [Google Scholar] [CrossRef] [PubMed]
- Dabire, K.R.; Diabate, A.; Djogbenou, L.; Ouari, A.; N’Guessan, R.; Ouedraogo, J.B.; Hougard, J.M.; Chandre, F.; Baldet, T. Dynamics of multiple insecticide resistance in the malaria vector Anopheles gambiae in a rice growing area in South-Western Burkina Faso. Malar. J. 2008, 7, 188. [Google Scholar] [CrossRef]
- Niang, E.A.; Konate, L.; Diallo, M.; Faye, O.; Dia, I. Patterns of insecticide resistance and knock down resistance (kdr) in malaria vectors An. arabiensis, An. coluzzii and An. gambiae from sympatric areas in Senegal. Parasit. Vectors 2016, 9, 71. [Google Scholar] [CrossRef]
- Sougoufara, S.; Thiaw, O.; Cailleau, A.; Diagne, N.; Harry, M.; Bouganali, C.; Sembene, P.M.; Doucoure, S.; Sokhna, C. The Impact of Periodic Distribution Campaigns of Long-Lasting Insecticidal-Treated Bed Nets on Malaria Vector Dynamics and Human Exposure in Dielmo, Senegal. Am. J. Trop. Med. Hyg. 2018, 98, 1343–1352. [Google Scholar] [CrossRef] [PubMed]
- Trape, J.F.; Rogier, C.; Konate, L.; Diagne, N.; Bouganali, H.; Canque, B.; Legros, F.; Badji, A.; Ndiaye, G.; Ndiaye, P.; et al. The Dielmo project: a longitudinal study of natural malaria infection and the mechanisms of protective immunity in a community living in a holoendemic area of Senegal. Am. J. Trop. Med. Hyg. 1994, 51, 123–137. [Google Scholar] [CrossRef] [PubMed]
- Fontenille, D.; Lochouarn, L.; Diatta, M.; Sokhna, C.; Dia, I.; Diagne, N.; Lemasson, J.J.; Ba, K.; Tall, A.; Rogier, C.; et al. Four years’ entomological study of the transmission of seasonal malaria in Senegal and the bionomics of Anopheles gambiae and A. arabiensis. Trans. R. Soc. Trop. Med. Hyg. 1997, 91, 647–652. [Google Scholar] [CrossRef] [PubMed]
- Fontenille, D.; Lochouarn, L.; Diagne, N.; Sokhna, C.; Lemasson, J.J.; Diatta, M.; Konate, L.; Faye, F.; Rogier, C.; Trape, J.F. High annual and seasonal variations in malaria transmission by anophelines and vector species composition in Dielmo, a holoendemic area in Senegal. Am. J. Trop. Med. Hyg. 1997, 56, 247–253. [Google Scholar] [CrossRef] [PubMed]
- Sokhna, C.S.; Diagne, N.; Lochouarn, L.; Rogier, C.; Trape, J.F.; Spiegel, A.; Fontenille, D. [Comparative evaluation of the plasmodial infection of Anopheles using ELISA and dissection. Consequences for the estimation of the transmission of malaria in 1995 in Ndiop, Senegal]. Parasite 1998, 5, 273–279. [Google Scholar] [CrossRef]
- Trape, J.F.; Tall, A.; Sokhna, C.; Ly, A.B.; Diagne, N.; Ndiath, O.; Mazenot, C.; Richard, V.; Badiane, A.; Dieye-Ba, F.; et al. The rise and fall of malaria in a West African rural community, Dielmo, Senegal, from 1990 to 2012: A 22 year longitudinal study. Lancet Infect. Dis. 2014, 14, 476–488. [Google Scholar] [CrossRef]
- Wotodjo, A.N.; Diagne, N.; Gaudart, J.; Richard, V.; Raoult, D.; Sokhna, C. Malaria risk factors in Dielmo, a Senegalese malaria-endemic village, between October and November of 2013: A case-control study. Am. J. Trop. Med. Hyg. 2015, 92, 565–568. [Google Scholar] [CrossRef]
- Gillies, M.T.; De Meillon, B. The Anophelinae of Africa, south of the Sahara; Publication of the South African Institute for Medical Research: Johannesburg, South Africa, 1968; Volume 54, 343p. [Google Scholar]
- Diagne, N.; Fontenille, D.; Konaté, L.; Faye, O.; Lamizana, M.T.; Legros, F.; Molez, J.F.; Trape, J.F.; Les Anophèles du Sénégal. Liste commentée et illustrée. Bull. Soc. Path. Exot. 1994, 87, 267–277. [Google Scholar]
- Detinova, T.S. Méthodes à Appliquer pour Classer par groupes d’âge les Diptères Présentant une Importance Médicale, Notamment Certains Vecteurs du Paludisme; Série de monographies; OMS: Geneva, Switzerland, 1963; Volume 47, 220p. [Google Scholar]
- Morlais, I.; Poncon, N.; Simard, F.; Cohuet, A.; Fontenille, D. Intraspecific nucleotide variation in Anopheles gambiae: new insights into the biology of malaria vectors. Am. J. Trop. Med. Hyg. 2004, 71, 795–802. [Google Scholar] [CrossRef]
- Wilkins, E.E.; Howell, P.I.; Benedict, M.Q. IMP PCR primers detect single nucleotide polymorphisms for Anopheles gambiae species identification, Mopti and Savanna rDNA types, and resistance to dieldrin in Anopheles arabiensis. Malar. J. 2006, 5, 125. [Google Scholar] [CrossRef] [PubMed]
- Huynh, L.Y.; Sandve, S.R.; Hannan, L.M.; Van, E.M.; Gimnig, J.E. Fitness costs of pyrethroid insecticide resistance in Anopheles gambiae. In Proceedings of the Annual Meeting of the Society for the Study of Evolution, Christchurch, New Zealand, 16–20 June 2007. [Google Scholar]
- Painter, I. GWASExactHW: Exact Hardy-Weinburg Testing for Genome Wide Association Studies. R Package Version 1.01. University of Washington, 2013. Available online: https://CRAN.R-project.org/package=GWASExactHW (accessed on 5 May 2024).
© 2024 Copyright by Authors. Licensed as an open access article using a CC BY 4.0 license.